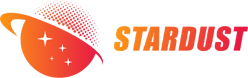
Tantalum is widely used in the field of medical implant materials due to its excellent biocompatibility, corrosion resistance and good mechanical properties [1-2]. Among them, the shape of porous tantalum is similar to the structure of human bone trabeculae, which is known as trabecular metal, and is characterised by a low modulus of elasticity, which is between human cortical bone and cancellous bone, and the modulus of elasticity that is close to that of the human bone tissues can effectively reduce the stress shielding effect in the human body [3-5]. The porosity of porous tantalum is in the range of 75%~80%, and the high porosity gives it good permeability and higher potential for inward tissue growth, which is conducive to osseointegration [6-7]. Porous tantalum is currently used in the fields of femoral head necrosis, joint replacement, and bone defects [8-9].
Tantalum's highly stable chemical properties (biologically inert) can delay its effective bonding with bone tissue and may be the main cause of implant loosening [10]. Therefore there is a need for surface modification of tantalum to improve its bioactivity. Methods to improve the surface activity of biomaterials include introduction of active elements [11-12] and improvement of surface roughness [13-14]. Among them, micro-arc oxidation (MAO), as an important surface modification method, can improve the surface roughness as well as introduce active elements conveniently [15-16]. For example, Li et al [17] successfully introduced active elements Ca and Sr into the coating while preparing a porous and rough TiO2 coating on the surface of pure titanium using micro-arc oxidation, and demonstrated that this coating improved the adhesion and spreading properties of osteoblasts on its surface. In addition, the micro-arc oxidation coatings characterised by in-situ growth have good bonding with the substrate, high wear resistance as well as corrosion resistance, excellent designability of the coating function and structure, and the ability to handle the inner and outer surfaces of complex devices [18-20].
In the preparation of implants, reducing the difference between implants and human natural bone can enhance the effect of bone implantation, and the use of bionic principles to construct micro- and nano-graded structures is one of the current trends. Micro/nano-graded structures can regulate the adhesion, proliferation, and differentiation of osteoblasts and facilitate the expression of osteogenic genes [21-22]. It has been demonstrated that micron-scale structures on the surface of the implant that are comparable to the cell size can enhance the contact area between the cells and the implant, affecting cell adhesion and spreading, which in turn affects cell proliferation as well as osteogenic differentiation ability [23]. Nanoscale structures, on the other hand, correspond to the dimensions of proteins and bone minerals, and surface nanoscale structures can increase protein adhesion sites, which in turn directly enhance cell adhesion, proliferation, and differentiation [24]. The microarc oxidation surface modification method allows the preparation of coatings with micrometre-scale grooves combined with submicrometre and nanometre-scale pores on the surface of pure titanium, which have likewise been shown to have excellent osteogenic differentiation as well as osseointegration capabilities in subsequent biological experiments [25].
Work has also been reported on the micro-arc oxidation of pure tantalum surfaces to enhance their bioactivity.Cheng et al [26] performed micro-arc oxidation of pure tantalum using silicate and introduced Si into the coating to enhance the bioactivity of the material surface.Wang et al [27] performed micro-arc oxidation of calcium acetate and β-glycerophosphate in calcium acetate and β-glycerophosphate, doping the coatings with Ca and P, and investigating the coating morphology, growth behaviour, etc., with the time of micro-arc oxidation, as well as the growth behaviour. behaviour, etc. with microarc oxidation time. Li Zhenzong et al [28] carried out microarc oxidation of porous tantalum in silicate as well as phosphate systems, followed by alkali treatment. The results showed that the composite treatment resulted in excellent biocompatibility and osteogenic properties due to the introduction of hydroxyl functional groups on the Ta2O5 surface.
The above coating structures obtained for pure tantalum micro-arc oxidation are mostly single-stage ‘crater’ structures, and the research focuses on how to introduce reactive elements or reactive groups on their surfaces, while less attention has been paid to the effect of different micro-arc oxidation morphology structures on their activities. In our recent work, we have successfully used tetraborate electrolyte to prepare a ‘bone-like trabeculae’-like porous hierarchical coating on the surface of pure tantalum. In order to investigate the effect of this biomimetic coating on the bioactivity of pure tantalum and to compare the differences in cytocompatibility between different morphologies, this paper compares and analyses the coating with that of ‘crater’-like structure on the surface of polished pure tantalum using the surface of polished pure tantalum as a reference. In order to investigate the effect of this biomimetic coating on the bioactivity of pure tantalum and to compare the differences in cytocompatibility between the different morphologies, we compare and analyse the differences in phase composition, coating bonding, roughness and hydrophilicity between the pure tantalum polished surface and the ‘crater’ micro-arc oxidized surface, and we also characterize the ability of the mouse preosteoblasts to spread, proliferate, and osteoblastically differentiate on the three surfaces.
1 Materials and methods
1.1 Sample preparation
Tantalum rods were cut into φ10mm×2mm tantalum sheets by wire cutting and polished cleanly using #200, #400, #800 and #1000 sandpaper in turn, and then ultrasonically cleaned using acetone, alcohol and deionised water respectively. The power supply used for micro-arc oxidation was a bipolar pulse power supply (WHD-20, Harbin Institute of Technology). The experiment used cleaned tantalum sheet as anode and stainless steel container as cathode. The electrolyte was 0.1 mol/L Na2B4O7, and the corresponding sample was named B-MAO. The power supply parameters were set to constant current 4A, duty cycle 9%, and frequency 600 Hz. In order to compare the effects of different micro-arc oxidation morphologies on the cytocompatibility of the samples, the sample (P-MAO) prepared with 0.05 mol/L Na3PO4 electrolyte was selected as the control group. The power supply parameters were set to constant current 12 A, duty cycle 9%, and frequency 600 Hz. mechanical stirring was used to dissipate heat during the reaction.
1.2 Characterisation of coating morphology and physical phase
The surface as well as the cross section of the coatings were analysed using a scanning electron microscope (SEM, Supra55, Zeiss, Germany). The phase structure of the coatings was determined using an X-ray diffractometer (XRD, Empyrean, PANalyticalB.V, The Netherlands) with Cu-Kα irradiation (λ = 0.154056 nm) at 40 kV with a step size of 0.04° and a scanning (2θ) range of 20° to 80°. The chemical valence states of the coatings were analysed using X-ray photoelectron spectroscopy (XPS, ESCALABXI+, Thermo, UK).The XPS tests were carried out using an Al-Kα radiation source (hυ = 1486.6 eV).Prior to the test, the surface of the samples was etched by an argon ion beam for 1 min to reduce possible carbon contamination, and C1s (284.8 eV) was used as a reference peak to calibrate All energy values. the XPS results were fitted by XPSpeak 4.1 software for peak splitting.
1.3 Coating adhesion test
The bond between the micro-arc oxidised coating and the substrate was determined using a cross-hatched adhesion tester using the ASTM method (ASTMD3359-B), which is applicable to metallic substrates. The first step is to make an incision on the surface of the coating with uniform pressure in two orthogonal directions, ensuring that the depth of the incision reaches the surface of the substrate. After making the cuts, a soft brush is used to remove the flakes from the surface of the coating. The coating adhesion rating was assessed by comparing the rating standards with the ASTM method and the surface morphology of the cuts was recorded using SEM.
1.4 Surface roughness and hydrophilicity testing
The 3D stereo features of the sample surfaces were measured using a 3D laser confocal scanning microscope (CLSM, OLS4000, Olympus, Japan) and the roughness of the coated surfaces was measured under a 20x objective lens. The water contact angle of the samples was measured using a contact angle meter (DSA100, KRÜSS, Germany) using a 2 μL water droplet, selected for 240 ms after the droplet contacted the coating. Five samples each were selected for the experiment, and five points on the sample surface were tested respectively, and the points with large differences were rounded off, and then the average value was calculated.
1.5 Cytocompatibility test
1.5.1 Cell culture
In this test, mouse preosteoblasts (MC3T3-E1, Cell Bank of the Chinese Academy of Sciences) were cultured by direct contact, and the medium used was α-MEM complete medium, which was prepared from α-MEM medium (Soleberg Technology Co., Ltd., Beijing, China) with the addition of 10% foetal bovine serum (FBS) and 1% double antibody (100 U/mL penicillin, 0.1 mg/mL streptomycin sulphate) The cell culture incubator (HERAcell) was prepared by adding 10% fetal bovine serum (FBS) and 1% double antibody (100 UL penicillin, 0 mg/mL streptomycin sulfate). The cell culture incubator (HERAcell150i, ThermoScientific, Germany) was maintained at a constant temperature of 37°C and a gaseous environment containing 5% carbon dioxide, and the complete medium was changed every 2-3 d during cell culture, and the cells were grown to about 80% of the culture flasks for cell passaging.1.5.2 Sample preparation
Sterilise the micro-arc oxidised and polished samples. Firstly, the samples were ultrasonically cleaned for 15min, then the cleaned samples were autoclaved at 120℃ for 30min, and then put into the vacuum drying oven for drying, and the dried samples were irradiated under ultraviolet light for 12h on both sides respectively, and the sterilised samples were put into the aseptic operating table for spare.
1.5.3 Cell spreading
Cells were inoculated onto the coated surface of the samples at a density of 2×104/mL, and incubated in a carbon dioxide incubator for 1, 2 and 4 h. The cytoskeleton was stained using fluorescein with ghost pen cyclic peptide (FITC, Biyuntian Biotech Co., Ltd., Shanghai, China), and the stained cells showed green fluorescence of F-type actin, and then the cells were observed by a fluorescence microscope (BX51, Olympus, Japan). The observation of the initial spreading of cells was carried out. Cells were inoculated onto the surface of the samples at a cell density of 2×104/mL, incubated in a carbon dioxide incubator for 2, 4, and 24 h, respectively, and then fixed using 4% paraformaldehyde, dehydrated using graded concentrations of ethanol (mass fractions of 30%, 50%, 70%, 80%, 90%, and 100%, respectively), and then dried at room temperature, and then the coatings were observed using a SEM structure on cell spreading.
1.5.4 Cell proliferation
Cells were inoculated onto the surface of the sample coating at a density of 2×104/mL, and after incubation for 1, 3, and 5 d, a cell counting kit (CCK-8, Solebo Technology Co., Ltd., Beijing, China) was added, and incubated at a constant temperature for 3.5 h. Then the cultured liquid was transferred to a 96-well plate, and an enzyme labeller (SynergyH1, ThermoScientific, USA) was used to Its absorbance at 450 nm was detected. Absorbance had a linear relationship with cell number and activity.
1.5.5 Alkaline phosphatase activity (ALP activity)
ALP is a marker of early cellular osteogenesis and the magnitude of its activity is positively correlated with the amount of osteoblastically differentiated cells. The osteogenic differentiation ability was determined using the ALP assay kit (Biyuntian Biotechnology Co., Ltd., Shanghai, China). Cells were inoculated onto the surface of the samples at a density of 2×104/mL, and cultured in complete medium for 3d, then the medium was removed, and then continued to be cultured using osteogenic induction medium, which was changed every 3d. After 7 and 14 d of incubation, the cell lysate supernatant was incubated with ALP chromogenic solution for 30 min, and the standard and blank control were incubated separately for 30 min, after which the reaction was terminated by adding reaction termination solution, and then the absorbance at 405 nm was measured. The ALP activity was calculated from the absorbance.
2 Results and Discussion
2.1 Coating morphology analysis
The microscopic morphology of the surface and cross-section of the B-MAO and P-MAO coatings is shown in Fig. 1. It can be seen that the surface of B-MAO exhibits ‘bone-like trabeculae’ porous features (see Fig. 1a). The thickness of the ‘bone trabeculae’ is about 2 μm, and the ‘bone trabeculae’-like structures form large-sized micron-sized grooves (groove widths of 5-8 μm), and small-sized micron-sized pores (pore diameters of 2 μm or so) are also distributed inside the grooves. Combined with the cross-sectional morphology of the coating (see Fig. 1c), it can be seen that there are also more smaller-sized pores inside the coating, which suggests that the B-MAO coating has a hierarchical structural feature in the film thickness direction. In contrast, the surface morphology of the P-MAO coating (see Fig. 1b) shows typical ‘volcanic crater’ porous features, with the size of the holes around 3 μm, and a small number of interconnecting holes to form grooves, but the morphology and size of the holes are relatively homogeneous, and the cross-sectional morphology of the P-MAO coating (see Fig. 1d) shows typical micro-arc discharge channel features, with the discharge channels of smaller size. micro-arc discharge channel characteristics, and the region outside the discharge channel is less porous and denser compared to the B-MAO coating. From the morphology, the porosity of MAO coatings obtained using Na2B4O7 electrolyte on pure tantalum surfaces is higher than that of MAO coatings prepared using Na3PO4, and such an effect may be related to the strong dissolving effect of tetraborate electrolyte on the coating's micro-arc discharge products [25].
Fig. 1 Surface and cross-section morphology of the coatings
2.2 Coating phase analysis
The XRD spectra of B-MAO and P-MAO coatings are shown in Fig. 2, and for comparison, the XRD spectra of pure tantalum before micro-arc oxidation are also given in the figure. Comparison of the standard PDF cards shows that the main physical phase of the B-MAO and P-MAO coatings is Ta2O5 (PDF#71-0639). Because of the high porosity of the coatings and the deeper X-ray penetration depth, diffraction peaks of basal pure Ta (PDF#04-0788) appeared in the spectra of the 2 coatings.
The XPS analysis results of the coated surfaces are shown in Fig. 3. Among them, Figs. 3a-d show the XPS analysis results of B-MAO, Fig. 3a shows the total spectrum, and Figs. 3b, c, and d show the detailed spectra and split-peak fitting results of Ta4f, O1s, and B1s, respectively.There are two characteristic peaks of Ta4f at binding energies of 25.80 and 27.69 eV, with spin-split orbitals of 1.9 eV, which is consistent with that of Ta5+. Ta4f5/2 and Ta4f7/2 characteristic peaks, close to the binding energy of Ta2O5 [29], but with a certain degree of offset (see Figure 3b). The characteristic peak with a binding energy of 530.06 eV, on the other hand, agrees with the O1s binding energy of Ta2O5, which proves that Ta exists as Ta2O5 on the coating surface [30] (see Fig. 3c). It has been shown that an amorphous layer exists on the surface of microarc oxidised coatings [25], and here, the Ta4f peak position of Ta2O5 is shifted towards higher binding energy, probably due to the amorphous state of Ta2O5 formed on the surface of the coatings [31].The peak position with higher bonding energy in O1s (531.64 eV) corresponds to B2O3 [32], and the B1s peak position (191.52 eV) corresponds to B2O3, indicating that B exists as B2O3 in the B-MAO coating (see Fig. 3d). The B2O3 diffraction peaks were not detected by XRD in Fig. 2, and it is presumed that the B2O3 in the coating should come from the thermal decomposition of the Na2B4O7 electrolyte and exist in small amounts on the surface mainly in an amorphous form [25]. Figure 3e-h shows the XPS analysis results of P-MAO. Among them, Fig. 3e shows the total XPS spectrum, and Figs. 3f, g, and h show the detailed spectra and split-peak fitting results of Ta4f, O1s, and P2p, respectively.Ta4f also has two characteristic peaks at binding energies of 26.14 and 28.05 eV, with spin-splitting orbitals approximated to 1.9 eV, and there is a certain shift from the binding energy of Ta2O5 (see Fig. 3f), but considering that in the detailed map of O1s Ta2O5 (530.43 eV) characteristic peak can be fitted (see Fig. 3g), it can be determined that Ta exists in the form of Ta2O5 in the P-MAO coatings.The higher bonding energy in the O1s peak position (531.43 eV) corresponds to PO-, combined with the P2p at 133.78 eV peak position at 133.78 eV (see Fig. 3h), proves that P exists in the form of PO-.
This indicates that a small amount of Na3PO4 electrolyte was deposited on the coating surface in an amorphous state due to the rapid cooling of the liquid on the coating surface during the micro-arc oxidation preparation of the P-MAO coating.
Fig. 2 XRD spectra of B-MAO, P-MAO coating and pure Ta.
Fig. 3 XPS spectra of B-MAO and P-MAO coatings.
2.3 Coating bond strength test
The SEM morphology of the two coating cuts after the cross-hatching test is shown in Fig. 4. It can be seen that there is a large area of shedding on the cross-hatched part of the surface of P-MAO, which is the internal cracking of the coating, indicating that the coating bonding strength is poorer.The shedding phenomenon on the surface of B-MAO is not obvious, and the incision is relatively flush. According to the rating standard of ASTM method, the P-MAO shedding area is 15%~35%, and the rating reaches 2B, while the B-MAO scribing edge is smooth, and there is no coating shedding at the intersection point, and the rating reaches the highest level of 5B. The above facts show that the bonding force of B-MAO coating is better than that of P-MAO coating. Analysing the cross-sectional morphology of the coatings (see Fig. 1), it can be seen that there is a relatively thin dense layer in the inner layer of the two coatings at the bonding position with the substrate, and an intermediate loose layer with a lot of holes outside the dense layer. The dense layer at the interface between the substrate and the coating has a higher bonding strength with the substrate because it is grown in situ, which means that the possibility of cracking and thus spalling at the interface between the coating and the substrate is lower. Analysing the reason why the P-MAO coating is easy to crack from the inside: on the one hand, it may be related to the bonding strength of the coating and the load-bearing area, compared with the B-MAO coating, the middle loose layer of the P-MAO coating has larger holes, which makes the coating internal delamination phenomenon, and the load-bearing area shrinks, which leads to the peeling off of the coating inside the process of cross-hatch and large-scale peeling off; on the other hand, the high porosity structure of the B-MAO surface helps to release the micro arcs of the micro arcs of the coating. On the other hand, the high porosity structure of the B-MAO surface helps to release the thermal stress and volumetric stress generated during the micro-arc oxidation process, while the P-MAO surface has a lower porosity and a relatively dense coating, which is prone to form residual stresses during the cooling process of micro-arc oxidation, thus resulting in peeling due to the high internal stresses in the cross-hatching process [33].
Fig. 4 SEM morphology of P-MAO and B-MAO coating surface incisions after the cross-hatching test.
2.4 Different surface roughness and hydrophilicity
Suitable implant surface roughness promotes earlier and stronger osseointegration, a rougher surface is a potential for enhancing the contact area between the implant and the bone tissue as well as the inward growth of the bone tissue, and osteoblasts have a better ability to attach to a rougher surface [34-35]. The 3D profiles of the surfaces of the different samples measured using laser confocal microscopy are shown in Figure 5. The roughness measurements showed that Ra = 0.06 μm for mechanically polished pure tantalum, Ra = 1.50 μm for P-MAO coating, and Ra = 1.58 μm for B-MAO coating, indicating that the B-MAO and P-MAO surfaces are rough surfaces, which are higher than that of the mechanically polished pure tantalum, and that the ‘trabeculae-like’ porous structure on the B-MAO surface has better adhesion ability than that of the mechanically polished pure tantalum. The roughness of the ‘bone trabeculae-like’ porous structure on the B-MAO surface is higher than that on the P-MAO surface.
Increasing the hydrophilicity of the implant surface can effectively stimulate the interaction between the implant and the biological environment of the human body and improve the adhesion of proteins, cells, etc., thus promoting osseointegration [36-37]. Hydrophilicity can be expressed by the size of the water contact angle, and the smaller the contact angle, the better the hydrophilicity. The contact angles of Ta, P-MAO and B-MAO were measured to be 42.6°, 15.5° and 7.2°, respectively. It can be seen that the contact angle of B-MAO is lower than that of P-MAO, and the contact angle of both of them is obviously smaller than that of polished Ta, which indicates that the hydrophilicity of the coating is better than that of the polished pure Ta. The contact angle of B-MAO is less than 10°, which is super hydrophilic, and it indicates that the ‘trabecular-like’ porous structure on the surface of B-MAO has an important influence on the hydrophilicity of the coating. This indicates that the ‘bone-like trabeculae’ porous structure prepared on the surface of B-MAO has a certain promotion effect on the hydrophilicity of the coating. It has been shown that the contact angle varies with the surface roughness of the sample, which satisfies the Wenzel model in the ideal case: cosθm=rcosθγ, where: θm is the measured contact angle; θγ is the Young's contact angle of the material; and r is the roughness factor (the ratio of the actual solid-liquid contact area to the apparent solid-liquid contact area, with r>1, which is related to the magnitude of the roughness) [38]. The above theory implies that for surfaces that are intrinsically hydrophilic (Young's contact angle less than 90°), roughness has an amplifying effect on their hydrophilicity. Here, the 2 microarc oxidation coatings are composed of Ta2O5 with polarity, which is inherently hydrophilic, so the rough microarc oxidation coatings have significantly better hydrophilicity than the polished pure Ta, and the roughness of the B-MAO coatings is larger than that of the P-MAO coatings, so their hydrophilicity is theoretically superior. In addition, compared with the discrete pore structure of P-MAO, B-MAO's ‘bone-like trabeculae’ coating has obvious groove-like features on the surface, with more interconnected pores, and its strong capillary effect can further increase its hydrophilicity and make it reach superhydrophilic state.
2.5 Cytocompatibility of different surfaces
The fluorescence staining images of cells cultured on pure Ta, P-MAO and B-MAO surfaces for 1, 2 and 4h are shown in Fig. 6.At 1h, the cells on the three morphology surfaces were rounded, and the number of cells on the P-MAO and B-MAO coated surfaces was more than that on the Ta surfaces, which indicated that at the initial stage, the adhesion ability of the cells on the P-MAO and B-MAO coated surfaces was higher than that on the polished Ta.As the time increased, the cells gradually spread on the surface, and the cell morphology developed into polygonal shape and some cells had pseudopods appeared at 2 h. At 4 h, the cell pseudopods further expanded and the filamentous pseudopods increased. This indicates that the pre-pure Ta surface, P-MAO and B-MAO coatings have good cell spreading performance. Figure 6b shows the SEM morphology images of cell spreading, which can be observed to further compare the effect of surface structure on cell spreading and adhesion. in the SEM images of 2h culture, the number of B-MAO compared to P-MAO cell adhesion was higher, and at 4h, the morphology of B-MAO cells appeared to be significantly changed, with a higher number of pseudopods. At a longer time point of 24 h, the cell spreading on the B-MAO coated surface has been more adequate and the cell spreading ability of B-MAO coating is better than that of P-MAO coating and polished pure Ta surface.Fiedler et al [39] suggested that different surface structures cause different stresses on actin fibres on the cell membrane, which in turn have different spreading rates. In this paper, the B-MAO surface is more favourable to the extension and spreading of filamentous pseudopods than the P-MAO surface, which should be related to its unique ‘trabeculae-like’ hierarchical porous structure.
Fig. 5 3D morphology and water contact angle of different surfaces.
Fig. 6 Fluorescence staining and SEM images of cells cultured on Ta, P-MAO and B-MAO surfaces for different times.
The CCK-8 detection results of cells cultured on Ta, P-MAO and B-MAO coated surfaces for 1, 3 and 5d are shown in Figure 7. As can be seen from the results, the number of cells on all three morphology surfaces showed a significant increase with time, indicating that none of the three surfaces were cytotoxic. At 1d of cell culture, the cell proliferation ability of the three surfaces was ranked as B-MAO>P-MAO>Ta, with a statistically significant difference in cell proliferation between the P-MAO and Ta surfaces (p<0.05), and a significant difference between the B-MAO and P-MAO (p<0.01). at 3d, this difference continued to exist, although the difference between the B-MAO and P-MAO was slightly decreased (p<0.05). at 5d, B-MAO had the optimal cell proliferation capacity, which was significantly different from P-MAO and pure Ta (p-values less than 0.001 and 0.01, respectively). Although the cell proliferation capacity of pure Ta was better than that of P-MAO at 5d, there was no statistically significant difference between them (p>0.05). The reason for this phenomenon may be analysed to be that in the case of prolonged cell culture, cells were spread out on both the single-stage structure and the polished Ta surface, whereas under the obvious longitudinal graded structure formed on the surface of B-MAO, the cells had a tendency to grow inward, which was more conducive to the growth of cells on the coating. It has been shown that the morphology with large specific surface area and higher connectivity helps cell adhesion and proliferation [40], which is consistent with the results observed in this experiment.
Fig. 7 Cell proliferation ability test of Ta, P-MAO and B-MAO surfaces for CCK-8 assay at 1, 3 and 5 d (ns p>0.05,* p<0.05, ** p<0.01, *** p<0.001, n=3 )
The quantitative results of ALP activity on the surfaces of Ta, P-MAO and B-MAO after 7 and 14 d of cell osteogenesis induction are shown in Fig. 8. It can be seen that with the increase of time, the ALP activity of the cells on different morphology surfaces increased significantly, and there was no statistically significant difference between the ALP activity of Ta and P-MAO coated surfaces at 7 and 14 d. However, the average value of the P-MAO coated ALP was better than that of the pure Ta at 14 d. The ALP activity of the B-MAO coating at the 2 time points was optimal, and it was with a significant difference. It has been shown that surfaces with high roughness are favourable for cell differentiation [41], which is verified by the results in this paper. Comparison of B-MAO and P-MAO showed that the chemical composition of the two surfaces was similar, and the reason for the difference in ALP activity between the two might be the difference in their surface structures. Since ALP is a marker for early cellular osteogenesis, the above results could suggest that the porous hierarchical structure formed by the B-MAO coating promotes cellular osteogenic differentiation more than the single-level structure of P-MAO.
Figure 8 ALP activity on the surface of Ta, P-MAO and B-MAO after 7 and 14 d of osteogenesis induction in MC3T3-E1 cells (ns p>0.05,** p<0.01, n= 3).
3 Conclusion
(1) The ‘bone-like trabeculae’-like graded porous and ‘volcanic crater’-like single-stage porous coatings were prepared on pure tantalum surfaces using micro-arc oxidation and Na2B4O7 and Na3PO4 electrolytes, respectively, and the main component of the coatings was Ta2O5. The main component of the two coatings is Ta2O5.
(2) The micro-arc oxidation coatings have higher roughness and hydrophilicity than the mechanically polished pure tantalum surfaces, as well as higher cell adhesion and proliferation ability.
(3) Compared with the ‘volcanic crater’ coating, the ‘bone-like trabeculae’ porous coating has stronger bonding force, higher roughness and superhydrophilic properties, and excellent performance in cell adhesion, spreading, proliferation and osteogenic differentiation, which has great potential for application in the field of hard tissue replacement. It has great potential in the field of hard tissue replacement.
Stardust's spherical tantalum powders are specially developed for 3D printing and orthopaedic implant applications and have excellent biocompatibility and mechanical properties. Through the RF plasma spheroidisation process, the spherical tantalum powder produced has uniform particle size and good flowability, which is particularly suitable for manufacturing porous tantalum structures for 3D printing. With its high porosity and excellent osseointegration ability, porous tantalum is widely used in orthopaedic implants such as hip, knee and spinal implants.
Welcome to contact our technician Cathie Zheng:+86 13318326187