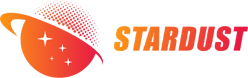
Refractory metal tungsten and its alloys have excellent properties such as high melting point, high hardness, high temperature strength, high modulus of elasticity, low coefficient of thermal expansion, good electrical conductivity and corrosion resistance, and they are widely used in cutting-edge fields such as aerospace, national defence and military industry, nuclear industry, medical equipment, electronics and so on [1]. Powder metallurgy is the most commonly used method to prepare tungsten and tungsten alloys. However, due to the brittleness of tungsten and its alloys at room temperature, some parts with complex structural features such as thin-walled, curved surfaces and porous parts are difficult to be prepared by conventional powder metallurgy, which limits the wider application of tungsten and tungsten alloys in the industrial field.
Additive manufacturing (additivemanufacturing, AM) is a new generation of ‘revolutionary’ manufacturing technology [2]. Compared with the traditional powder metallurgy method, AM has unique technical advantages such as high material utilisation, high manufacturing flexibility, small machining allowances, few structural design constraints, near-net shaping of complex structural parts, and fast response to structural design changes, etc., and has become one of the hot spots in the research of tungsten and tungsten alloys material preparation. At present, selective laser melting (SLM) and selective electron beam melting (SEBM) are two commonly used and studied AM technologies for the preparation of tungsten and tungsten alloys, and scholars at home and abroad have done a lot of research work on them. This paper briefly introduces the characteristics of SLM and SEBM technology, reviews in detail the current research status of SLM and SEBM in the preparation of tungsten and tungsten alloys, analyses the existing problems and shortcomings, and looks forward to the future development direction of SLM and SEBM in the preparation of tungsten and tungsten alloys, in order to provide a certain reference for the follow-up research on the preparation of refractory metal forming parts by SLM and SEBM.
1 SLM/SEBM technology
SLM preparation of metal forming parts is the use of laser on the metal powder based on the set path of rapid melting, layer by layer solidification and accumulation of forming parts of the preparation technology [3-4], the principle is shown in Figure 1 [5]. During the preparation process, an inert gas (high purity Ar or N2) is used to protect the metal from chemical reactions with other gases at high temperatures. The laser/powder interaction inputs a very high energy to the powder bed, which generates a large temperature gradient in the metal powder bed and produces physicochemical metallurgical processes such as heat transfer, mass transfer and phase transformation.SLM technology is used for the preparation of parts with complex shapes, especially those with complex internal cavity structures and those with personalised requirements, and is suitable for the rapid preparation of multi-species and small batch forming parts, which has a broad development prospect.
Fig.1 Schematic diagram of SLM technology[5]
Compared with SLM technology, SEBM uses high-energy electron beam as the heat source for powder spreading, preheating, and melting cycles to achieve the preparation of formed parts by layer-by-layer stacking [6]. During the preparation process, a high vacuum is maintained inside the chamber, which is suitable for the preparation of active metals and alloys that are sensitive to oxygen, nitrogen, etc. [7].SEBM technology has the features of high energy utilisation, high forming efficiency, low forming stress, and clean vacuum in the forming process, which is widely used in pure iron and steel, titanium and titanium alloys, nickel-based high-temperature alloys, cobalt-based alloys, copper and copper alloys, Co-Cr-Mo alloys, TiAl, and refractory metals, TiAl and refractory metals, etc. [8]. In addition, the powder preheating function of SEBM can keep the formed parts at a high temperature (up to 1000℃ or more), which has a significant effect on reducing the residual stress inside the parts, and is one of the advanced manufacturing technologies of titanium-aluminium-based alloys with a good prospect [9].SEBM improves the quality and efficiency of the preparation of the formed parts, and reduces the production cost. However, SEBM still has many problems, such as poor surface quality of the prepared formed parts, poor dimensional accuracy, etc., which still need to be further researched [10].Comparison of the characteristics of SLM/SEBM is shown in Table 1 [3, 6, 10].
Table 1 Comparison of SLM/SEBM characteristics
2 Research status of SLM/SEBM preparation of tungsten and tungsten alloy forming parts
In recent years, judging from the changes in the number of published research papers, the research on the preparation of tungsten and tungsten alloy formed parts by SLM and SEBM has shown a dramatic increase. Many countries and scientific research institutions have carried out a lot of research work on this, mainly in the United States, Germany, Britain, Japan and other countries, Tsinghua University, Nanjing University of Aeronautics and Astronautics, Central South University, Huazhong University of Science and Technology, Northwest Research Institute of Nonferrous Metals and other scientific research institutes, mainly focusing on the influence of raw material powder, forming process and alloying and other factors on tungsten and tungsten alloy molded parts, such as the densification, microstructure, mechanical properties and defects, etc. A large number of studies have been carried out. Research on the impact of raw material powder and alloying factors on the density, microstructure, mechanical properties and defects of tungsten and tungsten alloy formed parts, a large number of work is mainly concentrated in the pure tungsten, while the research on tungsten alloys such as W-Ta, W-Nb, W-Re, W-Y2O3, W-TiC, W-ZrC, etc. [11], as well as W-Ni-Fe alloys and so on, is relatively small (Fig. 2), and the technology of its preparation of formed parts is mainly concentrated in the SLM.
2.1 SLM preparation of tungsten and tungsten alloy formed parts of the current research situation
In the preparation of tungsten and tungsten alloys by SLM, the raw material powder, forming process, alloying and post-treatment have a great influence on the microstructure and mechanical properties of the formed parts.
2.1.1 Raw material powder is an important factor affecting the performance of tungsten and tungsten alloy formed parts prepared by SLM, and the powder morphology and particle size directly affect the interaction between the laser and the powder. Compared with irregular powder, due to the high density of spherical powder accumulation, when the laser interacts with the powder particles, the probability of absorption of the beam in the multiple reflections between the particles is also large. Studies have shown that the laser absorption rate of irregular powder is 50%, while the laser absorption rate of spherical powder is 68% [12].Zhang et al. [13] based on the results of line tracking simulation calculations show that: when the laser is acting on the powder particles, the multiple reflections of the laser beam between the powder particles strengthens the interactions between the neighbouring particles, which greatly facilitates the absorption of the laser light; however, with the increase of the powder particle size, the laser absorption rate of the powder layer decreases; the laser absorption rate of the powder layer decreases; the multiple reflections of the light beam between the particles also increase the probability of absorption. However, as the particle size of the powder increases, the laser absorption rate of the powder layer decreases; the maximum laser absorption rate of the tungsten powder layer is 0.6030 (tungsten powder particle size is 5μm).
Fig. 2 Types of tungsten and tungsten alloy materials prepared by SLM/SEBM technology
Several researchers at home and abroad have studied the effect of raw material powder morphology on the performance of pure tungsten formed parts prepared by SLM [12, 14-16].Wang et al. [12] obtained pure tungsten formed parts with densities of 84% and 96% respectively by using conventional powder and spherical powder as the raw materials as shown in Fig. 3.Zi et al. [14] also carried out the research in this area, and obtained the densities of 90.9% and 96.9% respectively. 90.9% and 96.9% respectively, as shown in Fig. 4. It can be seen that there are obvious holes and cracks inside the moulded parts prepared with irregular powder, while there are a few cracks and pores inside the pure tungsten moulded parts prepared with spherical tungsten powder. Figure 5 shows the densities of pure tungsten formed parts prepared by SLM using irregular and spherical powders as raw materials. Compared with the conventional tungsten powder, the spherical powder has a higher laser absorption rate, which is conducive to the formation of continuous scanning trajectory and the densification of the formed parts.Yamamoto et al. [15] used the irregular tungsten powder as the raw material to prepare pure tungsten formed parts with a densification of 98.58%, and Rebesan et al. [16] used the spherical tungsten powder as the raw material to prepare pure tungsten formed parts with a densification of 99.61%.
For this reason, many research institutions at home and abroad, such as the Huntsville Plasma Process Ltd. in the United States, Tekna Plasma Systems in Canada, the University of Science and Technology of Beijing and the Southwest Institute of Physics of the Nuclear Industry, etc., have carried out research work on the preparation of high-quality spherical tungsten powders, in order to meet the requirements of SLM on the performance of the powder.
Fig. 3 Morphology of pure tungsten moulded parts prepared from raw tungsten powder and SLM (SEM image)
Fig. 4 Morphology of pure tungsten moulding parts prepared by SLM (SEM image)
Fig. 5 Densities of pure tungsten formed parts prepared by SLM under different powder shape conditions.
2.1.2 Forming process
Adjusting the forming process to regulate the organisation and properties of the material is a common and important means for SLM to prepare formed parts, which can achieve the purpose of improving the material properties to a certain extent. Forming process mainly includes: forming process parameters, scanning strategy, substrate, chamber gas and other aspects.
(1) Forming process parameters
In the process of SLM preparation of formed parts, a number of process parameters together affect the final performance of formed parts. These process parameters mainly include: laser power, scanning speed, powder layer thickness and scanning spacing. Among them, Enneti et al [17] believe that the scanning speed is the main factor affecting the density of formed parts. At present, domestic and foreign scholars generally choose the input energy density to measure the size of the energy input in the preparation process of forming parts. Input energy density is mainly divided into line energy density (line energy densi⁃ ty, EL, J-mm-1) [18-20], areal energy den⁃ sity (ED, J-mm-2) [21-22] and volumetric en⁃ ergy density (EV) [21-22]. (J-mm-3) [5, 23-25], which are calculated as EL=P/v, ED=P/(v-h) and EV=P/(v-t-h), respectively. Where P is the laser power, W; v is the scanning speed, mm -s-1; t is the thickness of the layered powder layer, μm; and h is the scanning spacing, μm. However, most researchers often use EV to measure the input energy size. The relative densities of pure tungsten formed parts prepared by SLM under different energy densities are shown in Fig. 6, in which 85.7% of the research institutes have the relative densities of pure tungsten formed parts above 90%.
In the process of SLM preparation of formed parts, the laser energy output mode has continuous output mode and intermittent pulse mode. Nanjing University of Aeronautics and Astronautics and other [5, 20, 26, 34-35], Tsinghua University and other [12, 22, 28] research institutes have studied the effect of SLM process parameters on the performance of pure tungsten moulded parts by using continuous and pulsed laser output modes as the energy input source, respectively. In terms of experimental research, single pass is the most basic forming unit, and the final formed parts are essentially formed by stacking single passes, and the forming quality of single passes directly affects the quality of the final formed parts. Therefore, researchers generally use single-pass scanning to study the influence of each process parameter on the melt pool trajectory morphology, geometric features, defects, etc., to obtain the appropriate forming process parameter window, and initially realise the screening and optimization of the forming process parameters, and then carry out the solid part preparation based on the results of the single-pass scanning research. The single-pass scanning trajectory is an effective way to obtain the process parameter window for SLM preparation of formed parts.
Fig. 6 Relative densities of pure tungsten moulded parts prepared by SLM under different input energy conditions.
In terms of the continuous output mode of the laser, Gu et al. measured the input energy in terms of EL, and used the experimental method of single-pass scanning followed by solid part preparation to study the effects of laser power and scanning speed on the properties of formed parts, as shown in Figure 7 [34]. In terms of the surface characteristics of the scanning trajectory, a suitable EL can obtain a crack-free regular scanning trajectory (Fig. 7(b, b1)); whereas insufficient input energy results in an irregular liquid metal flow front, which causes the scanning trajectory to show obvious contraction phenomena, and also the presence of unmelted and splattering phenomena (Fig. 7(a, a1, g~i1)); and the input energy is too high resulting in the presence of a large temperature gradient of the liquid metal molten pool and the cooling rate, and crack defects will be generated on the surface of the scanning trajectory (Fig. 7 (c~e1)). In terms of scanning trajectory geometrical features, the increase in laser power and the decrease in scanning speed improve the EL, resulting in a decrease in the scanning trajectory height and contact angle, and an increase in the width and depth of the melt pool. In terms of the cross-sectional morphology of the scanning trajectory melt pool, the scanning trajectory melt pool was in a keyhole mode when the EL exceeded 1.5 J-mm-1, and was in a thermal conduction mode when the EL was lower than 1.5 J-mm-1 . Based on the results of the single-pass scanning study, the team obtained a pure tungsten solid part with a relative density of 98.4%, no obvious internal porosity and spheroidisation, with a microhardness and compressive strength of HV474 and 902 MPa, respectively, and a wear rate of 1.3 × 10-5mm3-N-1-m-1 [5].
In addition, Niu Punda et al [26] and Zhang et al [35] used SLM to prepare pure tungsten mouldings with fine grains. Niu Pengda et al. [26] prepared an equiaxial crystal former with grain size less than 1 μm (Figure 9), and the microhardness was HV485, which was much higher than that of the powder sintered samples, and Zhang et al. [35] prepared pure tungsten formers with a grain size of about 500 nm, and the fine grains grew perpendicular to each other under the condition of large laser energy input (Figure 10).
Wang et al. [12], Huang et al. [22] and Sidambe et al. [28] used pulsed output mode laser as the energy input source to carry out the research work, and the main factors affecting the pulsed mode are the laser power, exposure time, dot pitch and scanning distance, etc. Wang et al. [12] investigated the effects of laser power and exposure time on the surface morphology of pure tungsten moulded parts (Fig. 11). It was found that under different conditions of laser power and exposure time, dense regions, continuous regions and spherical regions appeared. At a spot spacing of 70 μm, the formed parts obtained a relative density of up to 96.0% and a hardness of 3.79 GPa. Spheroidisation was easily produced by either a small or too large input energy density. The authors concluded that the dot spacing and scanning distance are factors affecting the properties of the formed parts, and that both must be chosen to ensure the overlap of neighbouring scanning trajectories.
Fig. 7 Surface morphology of scanning trajectories of pure tungsten formed parts prepared by SLM under different input energy conditions (SEM images)
Huang et al [22] worked on the effect of scanning pitch and spot distance on the porosity of formed parts and prepared pure tungsten formed parts with a relative density of 92.5%. The apparent porosity is the unfused defects generated inside the formed parts under the condition of large scanning pitch and spot distance, which increases with the increase of scanning pitch and spot distance. Closed porosity is the spatter and keyhole caused by smaller scanning spacing and point spacing, which decreases with the increase of scanning spacing and point spacing. The porosity within the block is mainly dominated by apparent porosity.Sidambe et al [28] concluded that the laser energy density is sufficient for the preparation of pure tungsten shaped parts with densities up to 98%, which can satisfy the performance requirements of W-collimators and other plasma oriented materials.
Fig. 8 Properties of pure tungsten moulded parts prepared by SLM under different input energy conditions.
Fig. 9 Microstructure of pure tungsten prepared by SLM (SEM image)
Fig. 10 Microstructure of pure tungsten prepared by SLM under different scanning speeds (SEM image)
Fig. 11 Surface morphology of pure tungsten formed parts prepared by SLM under different process parameters (SEM image).
In addition, SLM has great advantages in the preparation of high value-added, small-lot and complex structure refractory metal formables.Deprez et al [37] used SLM to prepare pure tungsten collimator formables (Fig. 12) with a densification of 89.92% ± 0.5%, with dimensional accuracies ranging from -260 to 650 μm, average deviation of the aperture position of 5 μm, and average aperture size of (464 ± 19) μm, the sensitivity and resolution of the calculated and measured point sources at different positions in the field of view are in good conformity.Xie et al [38] also prepared pure tungsten gridded parts, the laser power has a large influence on the wall thickness of thin-walled parts, too high laser power will produce thermal cracks, and the optimisation of the process parameters can reduce the problem of powder adhesion on the surface of thin-walled parts.
(2) Scanning strategy
Scanning strategy is another important factor in the preparation of tungsten and tungsten alloy formed parts by SLM [39-40]. Higher energy input and uneven temperature distribution will cause large temperature gradient inside the melt pool, resulting in large residual stresses inside the formed parts, and even leading to warpage and deformation of the formed parts. The commonly used scanning strategies are: bi-directional scanning, island scanning and remelting scanning, with scanning vectors of no rotation, rotation 67. and rotation 90. (Table 2).
Fig. 12 Pure tungsten collimator formed parts
Gu et al [41] investigated the effects of island scanning, remelting scanning and bi-directional scanning strategies on the properties of pure tungsten formers prepared by SLM (Figure 13 and Table 3). The results show that the island scanning strategy is more prone to produce reticulated cracks due to discontinuous energy transfer and uneven energy distribution between islands. Meanwhile, between layers, the accumulation of inhomogeneous shrinkage during solidification leads to long cracks inside the formed part along the preparation direction, as shown in Fig. 13 (a1~a5). The remelting scanning strategy can reduce the internal porosity of the formed parts, reduce the residual stresses, and produce formed parts with good surface quality and fewer defects, but the remelting strategy will make the formed parts have coarse grains, which will adversely affect the mechanical properties of the formed parts, as shown in Fig. 13 (b1~b5). The bidirectional scanning strategy reduces the cracks of the formed parts to a certain extent due to the continuous energy transfer, even though the shrinkage of liquid metal solidification process is unavoidable, as shown in Fig. 13 (c1~c5). Based on the results of the above three scanning strategies, the mechanical properties of the formed parts prepared by the rotary 67. and bi-directional scanning strategies are the highest.
In the study of remelting scanning strategy affecting the performance of SLM-prepared pure tungsten formed parts, Xiong et al. [30] used a remelting scanning strategy with 1/2EV input energy to refine the internal grain of pure tungsten formed parts, which was mainly caused by remelting-induced convection of the liquid metal, which was in agreement with the view of Rebesan et al. [16] Zhou et al. [29, 51] concluded that the remelting strategy could weaken the mechanical properties of SLM-prepared pure tungsten formed parts. The remelting strategy can attenuate the spheroidisation phenomenon in the preparation of pure tungsten formed parts and improve the density of the formed parts. However, Xiong et al. [30] suggested that the remelting process may lead to the introduction of protective gases into the liquid metal flow, which may reduce the densities of the formed parts. In addition, the remelting strategy can reduce the residual stresses inside the formed parts, but it cannot completely inhibit the crack generation [30, 41].
Table 2 Scanning strategy of tungsten and alloy formed parts prepared by SLM technology (BD: forming direction; TD: transverse direction; SD: scanning direction)
Fig. 13 Morphology and organisation of pure tungsten prepared by SLM under different scanning strategies (SEM image) (laser power: 300 W, scanning rate: 300 mm s-1, layer thickness: 20 μm, scanning spacing: 50 μm).
Table 3 Comparison of tungsten moulded parts prepared by SLM under different scanning strategies.
Wang et al. [24] investigated the effects of two-way scanning strategies of no spinning, spinning 67, and spinning 67 with remelting on the cracks and microstructures of pure tungsten formed blocks prepared by SLM (Table 4). The results show that the scanning strategy of rotating 67. can make the grain boundaries entangled with each other and increase the crack extension resistance, which is conducive to the inhibition of crack extension.Wen et al. [19] and Zhou et al. [29] concluded that the scanning vector rotation of 67. between adjacent layers can reduce the internal residual stresses in the formed parts, and the prepared formed parts have fine grains without any obvious weaving.The experiments of Braun et al. [18] proved that the grain size of the formed parts is much finer than that of the formed parts made by rotating 90. between adjacent layers, compared with that of the formed parts made by rotating 90. between adjacent layers. Braun et al. [18] demonstrated that rotating 67 between adjacent layers is a better scanning strategy than rotating 90 between adjacent layers. Pure W has a high tendency to crack, and cracking is an unavoidable problem in the preparation of pure tungsten shapes by SLM, and the use of adjacent-layer rotational scanning can make the internal grain boundaries of the shapes intertwined with each other, which can help to inhibit the expansion of cracks.
Table 4 Comparison of tungsten forming blocks prepared by SLM under different scanning strategies.
Currently, the bi-directional scanning strategy with adjacent layer scanning vectors rotated 67. is the most widely used.
(3) Substrate aspect
Substrate is another factor that affects the performance of pure tungsten formed parts prepared by SLM. Substrate preheating, surface treatment and substrate material affect the temperature distribution and temperature gradient of the molten pool, which have an important influence on the residual stresses inside the formed parts.
In terms of substrate preheating, most of the substrates are preheated at low temperatures (below 200°C). Theoretically, the substrate preheating temperature is higher than the tough-brittle transition temperature (DBTT) of pure tungsten, and the screw dislocations have a high enough migration capacity to satisfy the plastic strain caused by the temperature gradient during the melting and cooling process.Ivekovic et al. [23] and Wang et al. [24] suggested that cracks inside the formed parts can be reduced by increasing the preheating temperature.Müller et al. [31] suggested that increasing the preheating temperature of substrates can improve the residual stresses inside the formed parts. Müller et al. [31] concluded that by increasing the preheating temperature of the substrate, the densities of the formed parts can be increased up to 98% (Figure 14). Kaserer et al. [18] concluded that the preheating temperature of the substrate up to 1000°C could not avoid the cracks in the tungsten moulded parts prepared by SLM.
In terms of substrate surface treatment, Zhou Xin and Liu Wei [51] treated the substrate with black paint to improve the absorption of laser light on the substrate. It was shown that the substrate with black paint-treated surface enhanced the laser absorption by the substrate/powder layer, which helped to increase the peak temperature of the molten droplet and prolonged the solidification time, and thus attenuated the tendency of pure tungsten molten droplets to sphericalise.
Substrate material affects the properties of formed parts by influencing the solidification rate of liquid metal. In terms of substrate material, most researchers choose stainless steel (thermal conductivity of 10.0-34.3 W-m-1-K-1) as the substrate, and Sidambe et al. [28] used pure titanium (thermal conductivity of 17.0 W-m-1-K-1) as the substrate for the preparation of pure tungsten (thermal conductivity of 163.3 W-m-1-K-1) formings, and the titanium reduced the cooling rate of the melt pool, so that the melt pool remained in the high-temperature stage. , so that the molten pool is kept in the high temperature stage for a longer period of time, which is favourable to the preparation of forming parts for shaping. Zhou, Xin and Liu, Wei [51] and Li et al. [43] chose pure tungsten substrates, and the subsequent processing can not consider the impurities introduced by the substrate [52-53].
Fig. 14 Relative densities of tungsten formed parts (substrate preheating temperature: 600.800 and 1000 °C; laser power: 375 and 400 W)
(4) For chamber gas
Argon (Ar) was chosen as the chamber gas by most researchers, while nitrogen (N2) was chosen by a few. dong et al [32] investigated the effect of chamber gas on the performance of pure tungsten formed parts prepared by SLM. Using Ar as the chamber gas, liquid metallic tungsten has high viscosity and is prone to spheroidisation and porosity, resulting in lower relative density and poorer mechanical properties of the prepared pure tungsten formed parts. Compared with Ar, using N2 as the chamber gas, W and N2 form tungsten nitride, which reduces the surface tension and viscosity of liquid metal, and improves the surface quality of the prepared pure tungsten formed parts, and the rapid cooling under N2 conditions also results in high hardness and strength. Therefore, the pure tungsten formed parts prepared by SLM under N2 conditions have higher density, lower surface roughness, and better mechanical properties (Table 5) [43], and the selection of N2 as the chamber gas is more favourable.Zi et al. [14] obtained pure tungsten formed parts with a density of 96.9% using N2 as the protective gas. In addition, Yamamoto et al [15] also believed that the internal nanoholes of pure tungsten moulding parts were caused by the retention of the protective gas Ar, so they could choose to prepare pure tungsten moulding parts under vacuum conditions, or choose N2 as the protective gas with smaller atomic size and higher diffusion coefficient.
2.1.3 Alloying aspect Due to the high surface tension, high viscosity and poor wettability of pure tungsten, the prepared pure tungsten forming parts have defects such as spheroidisation, porosity and cracks, which are the three most common defects in the preparation of pure tungsten forming parts by SLM. In addition to improving the raw material powder and forming process from the previous section, some researchers have added a certain amount of Ta, Nb, ZrC and Y2O3 into pure tungsten to improve the performance of pure tungsten formed parts.
(1) Generation of defects in pure tungsten forming parts prepared by SLM
Spheroidisation is one of the defects of SLM-prepared pure tungsten forming parts, and Zhou et al. [29, 51] took a 100-μm tungsten droplet as the object of study, and calculated that the liquid metal tungsten was fully spread at 6000 K (close to the boiling point of tungsten) for 86.3 μs, and the solidification time was 46 μs; in the vicinity of the melting point, the solidification time of the liquid metal tungsten was 7.6 μs, which makes the liquid metal tungsten in the process of solidification. The solidification time of liquid metal pure tungsten is 7.6 μs near the melting point, which makes liquid metal pure tungsten prone to spheroidisation during solidification.The spheroidisation of pure tungsten shaped parts prepared by SLM is shown in Fig. 15 [29]. In addition to the characteristics of tungsten itself, the spheroidisation phenomenon is also related to the oxygen in the material, which affects the surface tension and capillary convection in the liquid melt pool, further aggravating the tendency of spheroidisation.
Table 5 Properties of pure tungsten formed parts prepared by SLM under different chamber gas conditions
Fig. 15 Upper surface morphology of SLM-prepared pure W-formers (SEM image, exposure time 200 μs condition)
Cracking is one of the main defects in SLM-prepared pure tungsten formers, and the investigation of its cracking mechanism is a hot issue in current research [54-55].Cracks in SLM-prepared pure tungsten formers tend to expand along the large-angle grain boundaries, which is mainly due to the sensitivity of the grain boundaries to impurities in pure tungsten formers.Kaserer et al. suggested that the impurity oxygen is the main cause of the cracks and porosities in pure tungsten formers [18]. During the preparation process, oxygen is polarised at grain boundaries in the form of tungsten oxides, which weakens the grain boundary bonding strength, increases the risk of cold cracking, and raises the DBTT of pure tungsten.The combination of hot cracking at planar solidified grain boundaries and cold cracking along the weakened grain boundaries during rapid cooling creates the cracking network that is prevalent in pure tungsten shapes prepared by SLM.Vrancken et al. [36] suggested that microcracking occurs in a narrow temperature interval between 450~650 K. The cracks are also found to occur at the temperature of the grain boundaries. 650 K. A linear relationship exists between the time delay between solidification and cracking and P/v in the narrow temperature interval between 650 K and 650 K. The time delay between solidification and cracking is also a function of P/v.
Porosity is another common form of defect in formed parts [19]. Most of the reasons for the formation of porosity are attributed to process parameters, raw material powder and chamber gas. In terms of process parameters, insufficient energy input and large scanning spacing can cause the formation of unfused pores; too much energy input can cause the liquid metal to evaporate, and the vapour can not escape in time during the cooling and solidification process of the molten pool, which can produce round pores inside the formed parts. In terms of powder raw materials, the powder particles themselves are not dense, which is one of the reasons for the internal porosity of the formed parts. In addition, in the preparation of pure tungsten moulding parts by SLM, the chamber gas is easily caught in the melt pool and cannot escape in time, which is also prone to porosity. The porosity inside the formed parts affects their densities and mechanical properties, which can be reduced by optimising the process parameters, choosing suitable scanning strategies, alloying and post-treatment.
(2) Alloying
In order to eliminate and suppress the defects of SLM-prepared pure tungsten formed parts, Tsinghua University [25, 44], Xi'an Jiaotong University [45], Xi'an Air Force Engineering University [46], and KU Leuven University (Belgium) [23] and other research institutes have used to add a certain amount of Ta in pure tungsten to inhibit the generation of cracks in the internal parts of SLM-prepared pure tungsten formed parts.Wang et al. [25] believe that the formation of the tungsten oxide by the vaporization of the nanopores, which are mostly distributed at the grain boundaries of pure tungsten formed parts, are the main cause of crack initiation. After the addition of 6% Ta to W, there is a submicron cellular structure within the grains of W-6% Ta alloy, and the nanopores are mostly gathered at the cellular structure within the grains. Therefore, the authors concluded that the W-6%Ta alloy did not inhibit the formation of nanopores, but changed their distribution location (Fig. 16). However, compared with pure tungsten formed parts, cracks were effectively suppressed by 80% reduction in W-6% Ta alloy.Li et al [44] concluded that since the affinity of tantalum for oxygen is higher than that of tungsten, the addition of tantalum can adsorb the oxygen at the grain boundaries and form tantalum oxide nanoparticles (Ta0.8O2, Ta0.83O2, and TaO2) as the core of the heterogeneous nuclei, which can reduce the oxygen content at the grain boundaries and refine the grains, and improve the cracking resistance of the formed parts. grains, improve the resistance to crack extension of the formed parts, and reduce the crack density of the prepared W-6% Ta alloy by 30.7%.Ivekovi et al [23] concluded that the addition of Ta increased the hardness and recrystallisation temperature of W, reduced the thermal conductivity of pure W, and also facilitated the preparation of pure tungsten formed parts.
In addition, Yamamoto et al. [33] reduced the crack density by 59% by adding a certain amount of rhenium to W. Li et al. [43] reduced the crack density by 88.7% by adding 0.5% ZrC to W to refine the grains, reduce the concentration of oxygen impurities at the grain boundaries, and improve the strength of the grain boundaries, and Hu et al. [47] reduced the large-angle grain boundaries in the formed part by adding nanoscale 1% Y2O3. Hu et al. [47] reduced the large-angle grain boundaries in W, inhibited the crack growth and expansion of pure tungsten formed parts, and reduced the internal cracks of the formed parts, and Gu et al. [42] and Xue et al. [50] found that the addition of a certain amount of nano-sized TiC or Nb had a certain effect on the improvement and reduction of the internal cracks of pure tungsten formed parts, respectively.
Fig. 16 Electron channel liner imaging (ECCI) of nanopore distribution inside SLM-prepared pure tungsten and W-6%Ta formables
2.1.4 The suitable heat treatment can eliminate the residual stresses inside the formed parts, reduce the porosity and improve the performance of the formed parts. Zhang Menghan et al [21] heat-treated the pure tungsten formed parts prepared by SLM at 1400°C, which improved the flexural strength and microhardness of the formed parts.The related work of Ren et al [27] showed that the maximum tensile strength of the pure tungsten formed parts after annealing was 1.007 GPa.Yamamoto et al [15] believed that the nanoholes in the internal nanopores of the pure tungsten formed parts were formed due to the stagnation of the protective gas Ar, and after 1900°C×1000°C, the nanoholes in the pure tungsten formed parts were formed. After heat treatment at 1900°C×2h, the nanopores were reduced by 50% and the mechanical properties and thermal conductivity of the formed parts were improved. In contrast, the thin-walled formed parts of W-12% Ta alloy treated by Guo et al [46] using in-situ annealing process had a hardness value of 4.6 GPa and an elastic modulus of 179 GPa.
In addition, some researchers have also studied W-Ni [56], W-Ni-Fe [48-49], W-Ni-Cu [57] and W-Ni-Fe-Co [58] alloys prepared by SLM.Zhang et al. [56] used SLM to prepare W-Ni alloy formings with Ni contents of 10%, 20% and 40%, and the microstructures of these alloys were found to be very thin and thin, respectively, due to compositional Ni induced The microstructures of 90W-7Ni-3Fe (%, mass fraction) formed parts prepared by SLM, due to the different input of laser energy, the grains were transformed from cytosolic to dendritic in different regions [48].The microstructures of W-10Ni-10Cu (%, mass fraction) formed parts prepared by SLM, the amorphous W-Ni solid solution existed in the microstructure [59]. Ni solid solution [59].Li et al. [49] prepared 90W-7Ni-3Fe (%, mass fraction) formers with relative density ≥99%, which had a maximum tensile strength (UTS) of 1121 MPa, elongation <1%, and an average hardness of >HV0.3400.Iveković et al. [57] also heat-treated W-7Ni-3Fe (%, mass fraction) formers and compared with Li et al. Iveković et al [57] also heat-treated W-7Ni-3Fe (%, mass fraction) formers, and compared with the results obtained by Li et al [49], the tensile strength of the formers was reduced, while the elongation was improved.Chen et al [58] prepared W-Ni-Fe-Co formers by using SLM, and concluded that there was no significant effect of the laser parameters on the elongation of the W-Ni-Fe-Co.
2.2 Research status of SEBM for preparing tungsten and tungsten alloy formed parts
In recent years, SEBM has also been gradually applied to the preparation of complex structural forming parts due to its own advantages, and the material types include pure tungsten [60-65] and tungsten alloy [66-69]. At present, SEBM preparation of tungsten and tungsten alloy formed parts of the Institute of Northwest Nonferrous Metals Research Institute, Peking University, Northeastern University, etc., foreign countries, such as Germany, Germany, Ülich Research Centre, Oak Ridge National Laboratory in Tennessee, U.S., etc. SEBM preparation of formed parts is mainly affected by the melting current, the scanning speed, the thickness of the layer of laying powder and scanning spacing and other factors.
For the preparation of pure tungsten formed parts, Ren et al [60], Yang et al [61-62], Pengwei Yang [63], and Dorow-Gerspach et al [64] prepared nearly dense pure tungsten formed parts using SEBM, with the microstructure of coarse columnar crystals along the preparation direction. However, due to the high energy efficiency of the electron beam and the high thermal conductivity of tungsten, the substrate temperature was always kept at 800~950°C, which was conducive to the release of residual stresses inside the moulded parts.Ren et al. [60] prepared pure tungsten moulded parts with a relative density of 98.93%, with no cracks and a few porosities, with columnar crystals of 320 μm, grain orientation of <100>, and compressive strength of 1.76 Gbps using SEBM. Yang et al. [61-62] and Pengwei Yang [63] prepared pure tungsten formed parts with 99.5% densities and only a few internal cracks, with columnar crystals of about 200 μm in length and 50~150 μm in width, and <100> and <111> as the preferential orientation of columnar crystals in the forming direction, and the compressive strength of the formed parts was up to 1560 MPa, and the hardness of the formed parts was higher than that of the conventional ones, which was more than HV500, and the hardness was higher than that of the conventional ones. The compressive strength of the formed parts reached 1560 MPa and the Vickers hardness was above HV500, which was higher than that of the pure tungsten formed parts prepared by the conventional process.Dorow-Gers⁃pach et al [64] also prepared pure tungsten formed parts with a relative density of 99.5% and a width of columnar crystals of 100-200 μm by using the SEBM, and the hardness of the parts was about HV10350, which is close to the hardness of the recrystallised pure tungsten. The authors also concluded that in the SEBM process, it is not sufficient to consider only the effect of line energy density on the densities and quality of the formed parts, and the magnitude of the electron beam power should also be considered. Meanwhile, the experimental results also showed that the pure tungsten formed parts prepared by SEBM exhibited similar properties to those of conventionally recrystallised tungsten in terms of steady-state and transient thermal loads.Ellis et al. [65] also applied an in-situ defect detection algorithm to the detection of the internal porosity of pure tungsten in order to investigate the effect of the process parameters of the electron-beam powder-bed fusion (EB-PBF) on the defect density of the pure tungsten formed parts and concluded that higher preheating temperatures The authors concluded that a higher preheating temperature and a larger input energy can prepare dense, crack-free tungsten formed parts, and pointed out that the cracks inside the tungsten formed parts are mainly related to the oxygen content. In the future, further research can be carried out on the mechanism of the influence of energy density on the surface morphology of the formed parts and the grain refinement of pure tungsten formed parts prepared by SEBM with the addition of carbides or oxides.
In terms of tungsten alloys, Yang Guangyu et al [66] prepared W-3.5%Nb alloys using SEBM and investigated the effects of high-speed scanning, low-speed scanning and two scans on the microstructure of the formed parts and the defects of poor fusion and microcracking. The results showed that the poor fusion was mainly caused by the spheroidisation and disturbance of the melt pool, and the microcracks were mainly caused by the solidification shrinkage of the liquid phase between the dendrites during the solidification process. At high-speed scanning, due to the insufficient fusion between the scanning layers, the grain epitaxial growth is not obvious, leading to the formation of fine isometric crystals without obvious selective orientation, a large number of shrinkage holes on the surface of the melting layer, and a large number of poorly fused defects in the preparation direction. At low scanning speed, under the effect of epitaxial growth, a coarse columnar crystal organisation is formed with (001) optimal orientation along the forming direction, while shrinkage holes and poor fusion defects are significantly reduced. It was also found that the columnar crystal properties and the selective orientation of grains were weakened under two melting conditions in a single layer, but the fusion defects could not be completely eliminated and increased slightly compared to the low-speed scanning. Juntao Quan et al [67] concluded that the use of two different scanning paths to scan the metal powder layer successively will lead to a more uniform stress distribution in the part, which is less likely to lead to its warping and deformation, and can also improve the defects of poor fusion of the part to a certain extent.
In addition, Konyashin et al [68] also used SEBM to obtain dense WC-13% Co formed parts with upper surface roughness Ra = 4.4 μm and Rz = 22.8 μm (Ra is the arithmetic mean of the absolute value of the profile deviation within the sampling length; Rz is the sum of the average of the five largest profile peak heights and the average of the five largest profile valley depths within the sampling length ), the lateral roughness Ra = 24.5 μm and Rz = 142.3 μm, which is almost the same as the average size of the original WC-Co particles. The prepared shaped parts with 2% porosity can eliminate the porosity in the intermediate region after hot isostatic pressing (HIP).Xiao et al. [69] also prepared WTaRe refractory metal high entropy alloy with 98.5% densities by SEBM technique.
3 Summary and Prospect
The preparation of high-performance, complex structure of tungsten and tungsten alloy formed parts by AM technology has opened up a broader application field for tungsten and tungsten alloy. The main factors affecting the preparation of tungsten and tungsten alloy formed parts by AM are raw material powder, forming process (forming process parameters, scanning strategy, substrate, chamber gas), alloying and post-treatment. At present, although many researchers have carried out a lot of research in this area, there are still some problems that need to be further studied, mainly focusing on the following aspects: in terms of material system, the preparation of pure tungsten forming parts and the defect mechanism research is more in-depth and systematic, while the research on tungsten alloys is relatively small; in terms of preparation process, a large number of research work around the SLM, and not much research related to SEBM; in terms of material performance, the SLM forming parts are more in-depth and systematic, and there is less research on tungsten alloys. In terms of material properties, there are problems such as internal cracks have not been completely eliminated in SLM-formed parts, and the surface roughness of SEBM-formed parts is large, etc. In terms of numerical simulation, the intrinsic linkage of process parameters-microstructure-defect characteristics-mechanical properties has not yet been well established to quickly achieve the regulation and optimisation of the forming process, etc. Therefore, how to overcome and solve the above problems? Therefore, how to overcome and solve the above problems is still the focus and hot spot of future research on additive manufacturing of tungsten and tungsten alloy materials.
Reference:Research progress of tungsten and tungsten alloy materials prepared by additive manufacturing - Baidu Academic (baidu.com)
Stardust Technology adopts advanced RF plasma spheroidisation process to prepare spherical tungsten powders and tungsten alloy powders. These powders possess the following significant advantages:
High purity and low oxygen: through precise process control, the impurity content in the powder is extremely low, which ensures the high purity of the material and is suitable for demanding industrial applications.
High sphericity: The powder particles are highly spherical, contributing to more uniform deposition and higher finished product quality during the additive manufacturing process.
Controllable particle size: The particle size range of the powder can be adjusted according to specific needs to meet the requirements of different application scenarios.
Excellent flowability: The spherical particle structure and optimised particle size distribution ensure smooth flow of the powder in the additive manufacturing equipment, improving productivity.
These characteristics make Stardust's spherical tungsten powders and tungsten alloy powders ideal for high-end manufacturing fields such as additive manufacturing (e.g. 3D printing).
For more rare refractory metal spherical powders, please consult our professional technicians. Manager Zheng +86 13318326187.